Translate this page into:
Irradiation of blood components in combating transfusion associated graft versus host disease (TA- GvHD)
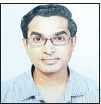
*Corresponding author: Dr. Anand Bodade, ICMR-CRMCH, Chandrapur under ICMR-NIIH, Mumbai, Maharashtra, India. anandbodade5@rediffmail.com
-
Received: ,
Accepted: ,
How to cite this article: Bodade A, Kumar I, Manii A. Irradiation of blood components in combating transfusion associated graft versus host disease (TA-GvHD). Med India. 2024;3:8–20. doi: 10.25259/MEDINDIA_35_2023
Abstract
Irradiation of cellular blood components is a specialized procedure performed to reduce one of the unusual but most serious adverse effects of transfusion therapy or transplantation, that is, transfusion-associated graft- versus-host disease (TA-GvHD). It is done using either gamma-rays or X-rays derived from radioactive sources. Various developed countries such as Great Britain, New Zealand, Australia, Canada, and Japan follow policies and guidelines for practicing transfusion of irradiated cellular blood components. In India, there are recommendations for the clinical use of irradiated blood components in indicated recipients. However, there is a lack of awareness about this specialized product, availability, usage, and its clinical importance. Furthermore, there are a few pros and cons of irradiating the blood components that need to be discussed. Most of the blood transfusion services departments or blood banks are not having in-house facilities of irradiation due to various reasons. There is a paucity of data published in literature based on clinical trials and evidences for the prevention of graft-versus-host disease by irradiation, and it mostly relies on case reports, hemovigilance data, and laboratory methods aiming to inactivate or eliminate lymphocytes in the transfused components. With this review article, we have made an attempt to describe the role of irradiation of blood components and its uses in the prevention of TA-GvHD, the different types of irradiators and methods of irradiation, dosimetry techniques, and the biochemical effect of irradiation on blood components. It will be helpful for peer clinicians to scrutinize the potential candidates for using irradiated blood components, considering its potential benefits.
Keywords
Irradiation
Blood components
Transfusion-associated graft-versus-host disease (TA-GvHD)
INTRODUCTION
Transfusion-associated graft-versus-host disease (TA-GvHD) was first reported in the 1960s in individuals with hematologic malignancies and in infants with congenital immunodeficiencies who developed “runting disease” after blood transfusion.[1]
TA-GvHD is a rare complication (<1 per million transfusions), and literature suggests it has a fatality rate of >90%.[2] There is no adequate estimate of prevalence worldwide. In Japan, the annual incidence of TA-GvHD is high, explained on the basis of homozygosity in the population for one-way human leukocyte antigen (HLA) haplotypes sharing, blood donors from family, and transfusion of fresh red blood cells (RBCs).[3] According to the US National Healthcare Safety Network, TA-GvHD is defined as fever, rash, hepatomegaly, or diarrhea occurring post-transfusion between two days and six weeks. Manifestations occurring within 100 days of post-transfusion or transplantation are termed as “acute graft-versus-host disease (aGvHD)” and those occurring after 100 days are called as “chronic graft-versus-host disease (cGvHD).”[4]
Graft-versus-host disease (GvHD) occurs after allogenic transfusion or transplantation due to the reaction of immune-competent cells of donor origin against the host tissues. The target organs damaged in GvHD mainly include bone marrow, skin, liver, and gastrointestinal tract (GIT). Skin manifestations include maculopapular rash, dyskeratosis, and exocytosis of lymphocytes. Diarrhea is one of the characteristic symptoms of GIT involvement. In severe conditions, it includes vomiting, anorexia, and abdominal pain. Liver involvement is manifested as increased levels of transaminases and histopathological changes in liver parenchyma. cGvHD is a pleiotropic, multiorgan syndrome involving tissue inflammation and fibrosis that can lead to permanent organ dysfunction. The manifestations of cGvHD are protean and often are of an autoimmune nature.[5-7]
PATHO-PHYSIOLOGY OF GvHD
In 1966–67, Billingham formulated the three requirements for GvHD to occur. These include:
The presence of immunologically competent cells in the graft tissue, that is, transfusion or transplantation of immune-competent donor lymphocyte cells.
A recipient incapable of rejecting the transplanted donor cells, that is, an immune-compromised host and,
The recipient must express human leukocyte antigens (HLA antigens) that are not present in the transplant donor that can be recognized as foreign by donor cells, that is, a disparity in histocompatibility in the donorrecipient pair.[8]
A wide range of host antigens can initiate GvHD, among them critical is the HLAs. There are certain protein molecules in HLA-identical siblings or unrelated donors with HLA- identical matches called minor-HLA. These are presented by major histocompatibility complex (MHC) molecules to the donor’s T-cells, which recognize them as foreign and mount an immune response. Thus, if the case of the donor is an identical twin sibling, GvHD can still occur. Antigens that are most responsible for graft loss are HLA-DR (first six months), HLA-B (first two years), and HLA-A (long-term survival).[9-11]
The pathophysiology of GvHD includes three phases [Figure 1]:
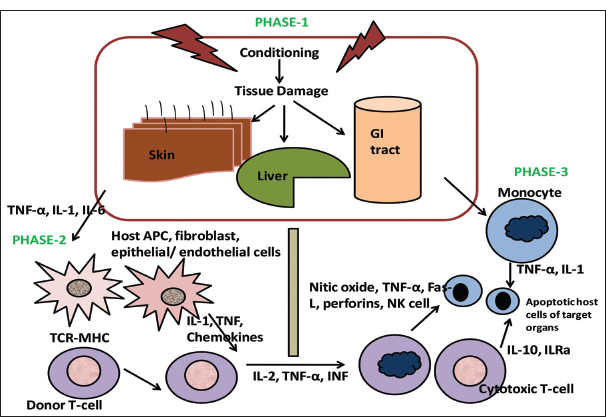
- Schematic representation of pathophysiology of graft-versus-host disease. TNF: Tumor Necrosis Factor-α, TCR: T-cell receptor, IL: Interleukin, NK: Natural Killer, ILRα: Interleukin-1 receptor family members, Fas-L: Fas Ligand
Afferent phase: Activation of antigen-presenting cells (APCs).
Efferent phase: Activation, proliferation, differentiation, and migration of effector cells.
Effector phase: Target tissue destruction.
The underlying pathology in the recipient, radiation therapy, and chemotherapy leads to the activation of host tissues and damage to the intestinal mucosa. This results in allowing microbial products to enter. They then stimulate proinflammatory cytokines, mainly interleukin-1 and tumor necrosis factor-alpha. These cytokines increase major histocompatibility complex (MHC) or HLA antigens expression and of adhesion molecules on APCs, ultimately increasing APCs’ ability to present antigens.[12] In the second phase, donor T-cells get activated and that enhances the expression of MHC and adhesion molecules, chemokines, and the expansion of CD8 + and CD4 + T-cells and guest B-cells.[13] As a result, these effector cells migrate to end-target organs and cause damage to tissue, resulting in targeted multiorgan failure.[14]
It mainly includes GIT mucosa, leading to diarrhea and liver damage which leads to hyperbilirubinemia. Skin manifestations include an erythematous rash on extremities and bone marrow suppression leading to pancytopenia and fever.
MANIFESTATIONS AND DIAGNOSIS
The aGvHD or cGvHD, whether due to allogenic transfusion/transplantation, follows similar pathophysiology and manifestations with minimal differences in their presentation. The following table compares the GvHD due to transfusion and transplantation [Table 1].[15]
Manifestation | HSCT GvHD | TA-GvHD |
---|---|---|
Median onset (range) | 23 days | 10 days |
(12–100 days) | (2–30 days) | |
Fever | Often | Usually |
Skin rash | + | + |
Liver involvement | ± | + |
GIT involvement | ± | ± |
Pancytopenia | Rare | Almost always |
Occurrence | 25–50% | Rare |
Response to therapy | 35–50% | Rare |
Mortality | 10–25% | 90–100% |
HSCT: Hematopoietic stem cell transplant, GvHD: Graft-versus-host disease, TA-GvHD: Transfusion-associated graft-versus-host disease, GIT: Gastrointestinal tract
Many different guidelines are available for aGvHD assessment for individual organ severity clinical staging, namely, Original Glucksberg,[16] Modified Glucksberg or Keystone criteria,[17] Mount Sinai aGvHD International Consortium[18] criteria, and International Bone Marrow Transplantation Registry criteria.[19]
As per the joint guidelines from the European Society for Blood and Marrow Transplantation (EBMT), the National Institutes of Health (NIH), and the Center for International Blood and Marrow Transplant Research (CIBMTR), GvHD is classified as follows depending on the manifestations in the patient [Table 2a]. For clinicians to take prompt action, the staging and grading of GvHD are imperative. EBMT 2019 criteria have proposed staging and grading of aGvHD that are presented in Table 2b and c, respectively, while cGvHD grading is outlined in Table 2d.[20]
aGvHD (classic or delayed onset) Skin – inflammatory maculopapular erythematous rash Liver - raised bilirubin levels GIT - anorexia, weight loss, nausea, vomiting, diarrhea, severe pain, GI bleeding, and/or ileus cGvHD (classic) manifestations meeting the NIH 2014diagnostic criteria, localized in Skin, nails, scalp, and body hair Mouth Eyes Esophagus Lungs Muscles, joints, and fascia Genitalia |
Undefined other cGvHD: Atypical signs and symptoms of alloreactivity falling outside the NIH 2014 diagnostic criteria Overlap cGvHD (encompassing manifestations of aGvHD and cGvHD) |
NIH: National Institutes of Health, GvHD: Graft -versus-host disease, aGvHD: Acute graft-versus-host disease, cGvHD: Chronic graft-versus-host disease, EBMT: European Society for Blood and Marrow Transplantation, GIT: Gastrointestinal tract, CIBMTR: Center for International Blood and Marrow Transplant Research
Stage | Skin based on maculopapular rash | Liver based on bilirubin | GI tract based on the quantity of diarrhea |
---|---|---|---|
+ | <25% of surface affected | 34–50 µmol/L | 500–1000 mL |
++ | 25–50% of surface affected | 51–102 µmol/L | 1001–1500 mL |
+++ | Generalized erythroderma | 103–255 µmol/L | >1500 mL |
++++ | Generalized erythroderma with bullae and desquamation | >225 µmol/L | Severe abdominal pain with/without ileus |
GI: Gastrointestinal, aGvHD: Acute graft-versus-host disease
Grade | Skin, GI, and liver stage | Decrease in clinical performance |
---|---|---|
I | Skin + - ++ | None |
II | Skin + - +++, GI, and/or liver + | Mild |
III | Skin stage ++ - +++, GI, and/or liver ++ - +++ | Marked |
IV | Skin stage ++ - ++++, GI, and/or liver ++ -++++ | Extreme |
GI: Gastrointestinal, aGvHD: Acute graft-versus-host disease, EBMT: European Society for Blood and Marrow Transplantation
Overall severity | Mild | Moderate | Severe |
---|---|---|---|
Number of involved organs | 1–2 | ≥ 3 | > 3 |
The severity of the involved organs | Mild (excluding lung) | Mild-moderate (lung mild) | Severe (lung moderate-severe) |
NIH: National institutes of health, cGvHD: Chronic graft-versus-host disease
Diagnosis of GvHD mostly relies on clinical presentation with supportive laboratory investigations, namely, pancytopenia in peripheral blood due to marrow suppression. Biopsy of skin, gut, or liver with characteristic histopathological changes is crucial. The presence of donor cells can be demonstrated by deoxyribonucleic acid (DNA) amplification in peripheral blood or short tandem repeat analysis using peripheral blood and skin biopsies from affected and non-affected sites in the patient, and peripheral blood samples from the implicated donors.[21] Fluorescence in situ hybridization, at times, is useful to diagnose TA-GvHD in sex-mismatched cases with rapid turnaround of results.[22] Recently, in 2018, “eGvHD application,” an electronic tool was launched to help clinicians classify GvHD based on the NIH-EBMT-CIBMTR criteria.[23]
INDICATIONS FOR IRRADIATION OF BLOOD COMPONENTS
-
Patients at risk of TA-GvHD or Recipients those who are potential candidates for irradiated blood components: [24-27]
Patients receiving transfusions:
From a first-degree relative (parents, children, or siblings) or second-degree relative (grandparent, grandchild, uncle, aunt, nephew, niece, or half-sibling)
Granulocyte concentrates
HLA selected components
Patients on purine analogs, for example, fludarabine, cladribine, deoxycoformycin, and bendamustine (irradiated blood components to be transfused until the availability of further data)
Intrauterine transfusions (IUT)
Red cell and/or platelet transfusions to neonates: Irradiated blood components should be given until six months of age in previously transfused neonates) or if the donor to recipient is a first or second-degree relative
Neonatal exchange transfusions (ET): if previous IUT was given or if the donor is a first/second- degree relative
For other neonatal ET, irradiation is recommended (care should be taken that the irradiation facility does not cause a significant delay in transfusion therapy)
Hodgkin’s Lymphoma, at any stage of the disease (for life)
Allogeneic hemopoietic stem cell (allo-HSC) graft from the start of conditioning therapy until the patient is on GvHD prophylaxis (6 months post-transplant). In the case of cGvHD, continue irradiated blood components indefinitely
Allogeneic HSC donors being transfused seven days before or during the harvest of their HSC.
Patients who will have autologous HSC graft in need of any transfusion:
Seven days before and during the bone marrow/stem cell harvest
From the day of conditioning chemo/radiotherapy until three months post-transplant (in case of total body irradiation, six months).
Aplastic anemia patients on immune-suppressive therapy with anti-thymocyte globulin and/or alemtuzumab (anti-CD52)
Known or suspected T-cell immunodeficiency, such as DiGeorge syndrome, the blood should be transfused within 24 h of irradiation
Receiving/received alemtuzumab for solid organ transplantation (for life)
Evidence is still under review for those recipients on T-cell depleted agents such as alemtuzumab for non-hematological indications such as multiple sclerosis and vasculitis.
-
As per the American Association of Blood Banks, clinical indications described are as follows [Table 3a].[28]
-
Certain additional clinical conditions: That might need irradiated blood components and need consensus clinical decisions based on evidence-based medicine include [Table 3b].[29]
Well-documented indications |
Intrauterine transfusions |
Premature, low-birth-weight infants |
New-born with erythroblastosis fetalis |
Congenital immunodeficiency |
Hematologic malignancies or solid tumors (Neuroblastoma, |
Sarcoma, and Hodgkin disease) |
Components that are cross-matched, HLA-matched, or directed donations |
Fludarabine therapy |
Granulocyte components |
Potential indications |
Other malignancies, including those treated with cytotoxic agents |
Donor-recipient pairs from genetically homogenous populations |
Usually not indicated |
Patients with human immunodeficiency virus |
Term infants |
Non-immunosuppressed patients |
HLA: Human leukocyte antigen, AABB: American Association of Blood Banks
Cytopenia from TBI exposure Cardiovascular surgery Recipient ≥age 65 years Massive blood loss of severe trauma Solid tumors undergoing treatment with high-dose chemotherapy or radiation Solid organ transplant: Lung, liver, heart, kidney, pancrease ECMO |
ECMO: Extracorporeal membrane oxygenation, TBI: Total body irradiation, EBM: Evidence-based medicine
CHOICE OF BLOOD COMPONENTS TO BE IRRADIATED
Transfusion of residual viable T-cells is necessary to develop TA-GvHD. As few as 5 x 104–1 x 105, T-cells per kilogram can mount GvHD in ablated hosts. Usually, a unit of packed red blood cells (RBCs) concentrate contains approximately 2 x 109 leukocytes, each whole-blood-derived platelet unit contains approximately 4 x 106 leukocytes, and apheresis platelets contain approximately 1 x 108 leukocytes. The leukocyte reduction procedure reduces the number of passenger T-cells by 2–3 logs, but it is not able to completely prevent TA-GvHD. A 2-log reduction of viable leukocytes can be achieved by washing red cells and a 2–3 log reduction is attained by freezing deglycerolization. For obvious reasons, leukocyte reduction is not performed with therapeutic white cell transfusions, and these can lead to TA-GvHD.[30]
After selecting potential candidates for irradiated blood components, one should know the kind of components that need irradiation. Usually, only cellular blood components, namely, red cells, platelets, and granulocytes, need to be irradiated. Fresh frozen plasma, cryoprecipitate, frozen washed red cells, and fractionated plasma products do not need to be irradiated as the lymphocytes will not or are extremely unlikely to survive the freezing/fractionation process. The different blood components that should be considered for irradiation purposes before they are transfused to the recipients are described in Table 4a. Red cells used for IUT/ET should be <5 days old when irradiated and transfused within 24 h of irradiation. This ensures optimal red cell function and minimizes the risk from free potassium (K+). Red cells for other patient groups need to be <14 days old when irradiated and will expire 14 days after irradiation.
Products known to contain viable T cells |
Whole blood |
PRBCs |
Frozen/deglycerolized RBCs |
Leukoreduced PRBCs |
Platelet concentrates, pooled |
Platelets-apheresis derived |
Granulocytes |
Non-frozen plasma (fresh plasma) |
HSCs |
Products that may contain viable T-cells |
Fresh frozen plasma |
Frozen plasma (e.g., FP24) |
Products unlikely to contain viable T-cells |
Cryoprecipitate |
SD-plasma |
“Pathogen-reduced” products (in development): PRT |
SD: Solvent detergent, PRT: Pathogen reduction techniques, HSCs: Hematopoietic stem cells, PRBCs: Packed red blood cells, RBC: Red blood cell, GvHD: Graft-versus-host disease
Red cells should be transfused within 24 h of irradiation in recipients with a risk of hyperkalemia as the K+ levels in irradiated units are high, approximately twice that of nonirradiated components. Washed red cells that are irradiated will expire at 23:59 h on the day following irradiation and can have a maximum shelf-life of 24–48 h (for an open system of saline washing, it will be 24 h). Platelets can be irradiated at any stage and stored up to their normal shelf life of five days or seven days. Granulocytes should be irradiated soon after they are collected and used without delay since their shelf life is 24 h from collection.[30]
PREVENTION AND TREATMENT OF GvHD
-
Principles of post-transfusion graft-versus-host disease (PT-GvHD) prevention in clinical practice: (ref: US- REMM)[29]
To avoid unnecessary transfusions.
To avoid transfusions from first-degree relatives whenever possible. If no alternate donor is available, use irradiated products from biological relatives.
Wherever possible, except for platelet concentrates, transfusion of freshly collected blood (<3 days) should be avoided.
Irradiation helps to prevent PT-GvHD in unavoidable allogeneic blood transfusions.
One should not wait for irradiating blood components in case, there is an urgent need for blood transfusion if irradiation is not available immediately.
There is a high risk of PT-GVHD if blood is fresh (<3 days), due to the presence of viable leukocytes.
It is observed that though leukocyte reduction by filtration is not sufficient to prevent PT-GvHD, it diminishes the chances of febrile non-hemolytic transfusion reactions, immunosuppressive effects of blood transfusions, platelet alloimmunization, cytomegalovirus infection, and other leucotropic viruses’ transmission and so it should be practiced.
If available, both irradiation and leukocyte reduction should be utilized.
To reduce the incidence and severity of GvHD, HLA/tissue typing should preferably be based on molecular techniques as it makes it more precise.[31]
Stem cells derived from umbilical cord blood contain T-cells with immunological immaturity that can reduce the incidence and severity of GvHD in transplantation.[32,33]
Use of GvHD prophylaxis, namely, methotrexate, cyclosporin, and tacrolimus.[34,35]
Use of mesenchymal stromal cells (MSCs) for prophylaxis needs further research.[36]
It is encouraged to use T-cell-depleted graft in bone marrow transplants. However, at times, it may lead to diminished graft-versus-tumor effect, risk of engraftment failure, cancer relapse, or general immunodeficiency. This results in more chances of infections, including opportunistic infections.[37]
-
Treatment of GvHD:
The use of IV glucocorticoids (e.g., prednisone) remains the mainstay as treatment in aGvHD and cGvHD to suppress T-cell-mediated immune onslaught on the host tissues. However, at high doses, it may cause immune suppression and risk of opportunistic infections, and cancer relapse[38,39]
Calcineurin inhibitors: Cyclosporin binds to the cytosolic protein peptidyl-prolyl cis-trans isomerase A (known as cyclophilin), and tacrolimus binds to the cytosolic protein peptidyl-prolyl cis-trans isomerase FKBP12. These complexes inhibit calcineurin, and they block dephosphorylation of the transcription factor, and nuclear factor of activated T cells from activated T-cells and its translocation into the nucleus.
Other drugs under trials for GvHD treatment: sirolimus, pentostatin, etanercept, and alemtuzumab.[40,41]
Recently, the US Food and Drug Administration (FDA) has approved ibrutinib for treating cGvHD after failure of ≥1 other systemic treatment.[42]
-
Recent advances:
Prochymal is a new drug approved by Canadian health regulators in 2012 for children with steroid- resistant aGvHD[43]
In a phase 2 clinical trial (a mesenchymal stem cell therapy known as remestemcel-L or MSC-100-IV) on 241 children with steroid-resistant aGvHD showed a survival rate of 82% versus 39% of control and promising results for long-term prevention of the same [Table 4b].[26]
Therapeutic approaches to decrease immune reactivity and tissue sensitivity | Therapeutic approaches to promote immune and tissue tolerance |
---|---|
T-cell suppression | Regulatory T-cells |
Anti-CD26 | Direct adoptive transfer |
Immunotoxin conjugated anti-CD3 and CD7 antibodies | Stimulators Extracorporeal photopheresis Post-transplant cyclophosphamide |
T-cell activation | Next-generation MSCs and MDSCs (Remestemcel-L) |
JAK1/2-STAT inhibition (Ruxolitinib, Itacitinib) Proteasome inhibitors IL-6 inhibitors (Tocilizumab) |
|
T cell migration | Stem cell repair |
CCR5 blockade (Maraviroc) | IL22 (F-652, Defibrotide) |
Integrin α4β1 blockade (Vedolizumab) | α1 anti-trypsin |
Sphingosine 1 receptor agonists (KRP203) |
MSC: Mesenchymal stromal cell, IL: Interleukin, GvHD: Graft-versus-host disease, MDSC: Myeloid derived suppressor cell
GUIDELINES IN CLINICAL TRANSFUSION PRACTICES
Recently, the guideline was proposed by the British Society for Hematology (https://b-s-h.org.uk/media/16732/bsh-guidance-development-process-dec-5-18.pdf).[44] The Grading of Recommendations Assessment, Development, and Evaluation (GRADE) nomenclature was used to evaluate levels of evidence and to assess the strength of recommendations. The GRADE criteria can be found at http://www.gradeworkinggroup.com. [45]
-
Dose of Irradiation:
It should be sufficient to inhibit residual T-lymphocyte in cellular blood components, without damaging the red cells, platelets, stem cells, and granulocytes. The response of the lymphocytes to allogeneic cells can be reduced by 5 Gy (Gray) of gamma (γ) irradiation and mitogen-induced stimulation can be reduced up to 90% using 15–20 Gy dose of radiation. A dose of 50 Gy can decrease the post-transfusion yield of platelets. It is found that the recommended dose varies from 15 to 35 Gy.[46] Various countries follow the dose of irradiation required for blood components preventing GvHD in clinical setting as described in Table 5.[47]
Sr. no. | Country | Dose of radiation |
---|---|---|
1 | India | 25 Gy±2 |
2. | USA | 25 Gy |
3. | Japan | 15–50 Gy |
4. | Great Britain | 25–50 Gy |
5. | Australia and New Zealand | 30–50 Gy |
6. | Canada | 15–50 Gy |
BLOOD IRRADIATION METHODS AND IRRADIATORS TYPES
Irradiation helps prevent TA-GvHD by inducing DNA cross-links of residual leukocytes in blood components thus preventing their proliferation. The recommended dose to component units is at least 25 Gy in the center and minimum delivered dose delivered to any other portion must be 15 Gy. Nowhere, the dose should go beyond 50 Gy where the mixed lymphocyte culture activity is abrogated. Various irradiators are used including gamma irradiation or X-rays. FDA-approved/cleared devices using gamma-rays from either Cesium-137 or Cobalt-60 sources or Devices using X-rays in use are model RS-3400 and Raycell Mk2.[48] It is highly recommended that all blood transfusion services (BTS) especially those who cater transplant services should have in-built irradiation facility. Following information can be helpful to the BTS/hospitals for installing the irradiators so that patients can get benefited in due course of time.
Gamma irradiators
Gamma irradiations are accomplished using Cesium-137 or Cobalt-60 sources.
Cesium Chloride
The natural occurring stable isotope of Cesium (Cs) is Cs- 135. A number of the radioactive isotopes of the Cesium are derived from Uranium or Plutonium by the process of nuclear fission. Cs-137 is usually used due to its long halflife. Cs-137 is used in devices in the form of cesium chloride. The cesium chloride irradiator is used in free-standing configuration. The Cs-137 source is placed in the form of pencil. The blood components to be irradiated are placed on the rotator turntable. The continuous rotation of the turntable allows the blood products to be irradiated by γ-rays from the pencil cells [Figure 2]. The speed of the rotator depends on the model of the instruments. CsCl2 has half-life of 30 years, which reduces transportation-associated risk.
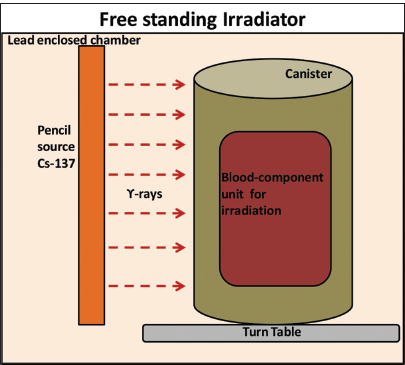
- Schematic representation of free-standing irradiator.
Cobalt-60 (Co60)
The Co60 is another commonly used source of gamma radiation. Co60 disintegrates into a stable Nickel isotope by emitting one negative β-particle. Co60 has a half-life of 5.27 years. During decay of Co60, two photons of the energy 1.17 and 1.33 Mev are emitted in succession to reach its stable state. These gamma radiations are used for the radiation process in the Co60 gamma irradiators. For irradiation, Co59 is used to convert it in Co60 in the nuclear reactor. Then slugs or pellets are made from this Co60. During the conversion of Co59 to Co60, a small percentage of the atoms in the slug are converted to Co60. The capsules containing slugs are encapsulated with corrosion-resistant stainless steel to produce source pencils. These source pencils are filled in the source modules.[49]
The gamma irradiators can be divided into two broad types: Self-contained irradiators and panoramic irradiators.
Self-contained irradiators
The self-contained are divided into two categories by International Atomic Energy Agency as Category-I (Dry storage) and Category-III (Wet Storage). In Category-I, the source of irradiation is placed inside a dry container surrounded by a protective shield of lead or some other suitable material. The source is distributed in the form of rings. The substance or material to be irradiated is driven to the source and is placed in between the sources so that material is irradiated from all the directions by the source.
These irradiators have a limited sample size of 1–5 L. This volume is adequate for research and scale-irradiations. In Category-III self-contained irradiators, the shielding is provided by water. In this, the source is motionless at the bottom of the tank filled with water. The water column has three functions: First, it acts as a radiation shielding, the second, it restricts the human access, the third is to dissipate the heat generated by the source, and fourth, it maintains the transparency of the source rack. The material to be irradiated is placed inside the steel containers, and the dose is determined by the dwell time of the containers.[50]
Panoramic irradiators
Similar to the self-contained irradiators, the panoramic irradiators are divided into two categories, that is, Category-II and Category-V. These irradiators are uses for the commercial purposes. In Category-II (Dry), the source is kept inside the shield when not in use. This irradiator allows human access to the irradiation room. While irradiation the source is hoisted from shield, extra shield to the irradiator is provided to protect humans from irradiation. In Category-IV (Wet), the water is used as shielding. There are two positions for the source rack: Shielded and exposed. In shielding, the source rack is placed several meters deep in the water tank. In exposed condition, the source is placed out of the tank to irradiate the material. The product containers go into tank and can travel at different levels. For low dose requirement, the container moves continuously and conveyor speed is adjusted to get a suitable dose and for high dose, the conveyor speed is kept low and the container dwells around the source. The dwell time selected on the basis of dose required.[51]
Radiation shield for gamma irradiators
As cesium chloride (CsCl2) is highly soluble in water, so it should not be used as source in wet storage gamma irradiators. A radioactive shield is generally built around the irradiation room. It is planned in accordance with safety standard for workers. Ordinary concrete is used for this purpose. For Co60 thicknesses from 1.5 to 2 m and for cesium chloride, the thickness of concrete wall can be reduced by about 0.5 m. The concrete wall is constructed as a corridor called maze so that there can be possible transportation of goods and movement of humans. To check whether there is radioactive leakage, a Geige-Muller counter can be used. Geiger Muller counter detects the ionization radiations such as α-radiations, β-radiations, and - rays using ionization effect produced in Geiger-Muller tube. A film badge can be attached outside the irradiator. Pulses can be monitored using the Geiger-Muller counter.[52]
X-ray irradiators
X-ray irradiators are based on non-isotopic sources. The X-rays can be emitted from the accelerated beam of the electron. The X-rays are designed in two forms: The first, a machine where a drawer holding blood bags is irradiated between the two X-rays tubes and the second, where the blood bags are placed on a rotator which moves in the full circle to ensure equal exposure from a single X-ray tube. The latter design is useful as it uses the majority of X-rays that normally absorbed in the X-ray tube itself [Figure 3]. The rotator increases the throughput and can irradiate more blood product in less time. The X-ray units require less security, eliminate liability, and not require expensive disposal at the end of machine’s life cycle.[53]
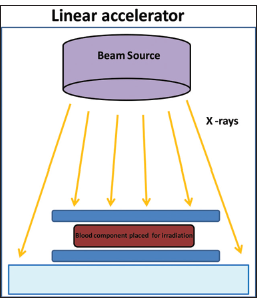
- Schematic representation of linear irradiator.
CsCl -137 versus alternative technologies
CsCl-137 is used as a source in gamma irradiator due to its longer half-life of 30 years, moderate level gamma-ray energy emission. The CsCl-137 can be used misused in spreading terrorism commonly known as radiological terrorism. This terrorism can result in a large number of fatalities. However, these risks can be reduced using alternatives such as Co60 and X-rays. However, the X-rays technique is more preferred over Co60 as the gamma-ray emission energy by Co60 is 4 times than that of Cs137, and hence, it requires more shielding than Cs137. The X-ray blood irradiators do not require any special requirements as Cs137 irradiators require for shielding. The cost of disposing X-ray blood irradiators at the end of its lifecycle is much lesser than CsCl-137 irradiators. The purchase cost of the X-ray is much lesser. X-ray irradiators require a large amount of electricity and water to cool down the X-ray tube. The replacement of CsCl2 with X-ray blood irradiators has positive benefits from both the private and social perspectives.[54]
DOSIMETRY
The dose of the irradiation can be measured by different types of dosimetry systems, namely, thermoluminescent dosimeters (TLDs), metal oxide semiconductor fieldeffect transistors (MOSFETs), chloroform/dithizone mixture, alanine/electron spin resonance (alanine/ESR), spectrometer, and radiochromic films. TLDs chips are small plastic chips. It has a few millimeters of dimension and has a crystal lattice that can absorb the radiations. The energy absorbed by these chips is measured for the dosimetry.
Another approach to irradiation dose mapping is MOSFETs. These transistors absorb and store the radiation electronically. Due to the radiation, there is a formation of holes in the metal oxide layer. The magnitude of the holes is measured by measuring the voltage with a voltmeter across the transistor. The measured voltage is used to evaluate the dose. Radiochromic films can be used in two ways. In the first system, the films get darkened, at a different part of the film after the exposure of the radiation, and the optical density of the film is increased. The absorbed dose of irradiation is calculated. It is found that absorbed irradiation is proportional to the optical density. In the second, one radiochromic having different radiationsensitive characteristics is used. This film is placed in the two halves of a circular-fitting phantom. The irradiation is performed with different time settings and the map produced is normalized to find the dose of irradiation. The absorbed dose is measured in cGy units. There is some degree of uncertainty or error in dose value when one uses different types of dosimetry methods. For most of the dosimetry techniques, the value of the uncertainty is 5% of the measured value.[55]
EFFECT OF IRRADIATION ON BLOOD COMPONENT
Irradiation leads to increased storage lesions that may have harmful effects if transfused. γ-irradiation causes detrimental changes in stored red cells viz. morphological, functional and metabolic changes collectively called as ‘storage lesions’ and they are sufficiently studied. As per U.S. FDA, irradiated RBCs be stored until the end of their shelf-life, but not more than 28 days from the date of irradiation. However, the Council of Europe advises that irradiated RBCs should not be processed within 14 days of collection.[56] Probably, it could be a wise decision in practice to irradiate the blood just before transfusion as suggested by Reverberi et al.[57]
Irradiation causes breaks in the DNA molecules of T-lymphocytes and prevents TA-GvHD. It may damage other cells such as red cells, platelets, and granulocytes simultaneously. It is found that the concentration of the K+ increases from 5.16 mmol/L to 28.60 mmol/L after irradiation in stored blood for 7–28 days. Normally, the Na+ concentrate is inversely proportional to K+ concentrate in red cells. After irradiation K+ value increases and Na+ concentration decreases. The pH of the blood changes after irradiation. The pH of the irradiated blood ranges from 6.39 to 7.07. The pH of the blood is influenced by the irradiation dose. There can be damage to the erythrocyte membrane due to irradiation and different doses of irradiation have different impacts on the erythrocyte membrane. During storage, irradiation on RBC leads to increased lactate dehydrogenase (LDH), hemolysis index, and potassium (K+). Although during storage irradiation affects quality, namely, changing parameters such as sodium, hemolysis index, LDH, and product specifications eventually are observed within limits of permissible quality control range.[58-60]
Ultrastructural damages to erythrocytes such as change of protein structure, alterations of deformability, cell membrane rupture, and modification of the permeability may be caused by Cs-137 irradiation. Other striking potential hazards of irradiation include radiation-induced malignant change, reactivation of the latent viruses, and leakage of plasticizers. When the dose of γ-irradiation exceeds the lethal dose of a cell, it leads to cell death rather than transformation. Moreover, it can possibly reactivate latent viruses. Another theoretical risk of irradiation includes leakage of plasticizer and entering into circulation of recipients of large-volume transfusions of irradiation components. It is advisable to follow the quality assurance of products and procedure as per available national guidelines and the standard operating procedures available in their institutes.[61-63] The parameters need to be considered for the same is depicted in Table 6.[64]
Dose | 25 Gy to the central midplane of a canister (freestanding irradiator) or to the center of an irradiation field (linear accelerator) with a minimum of 15 Gy |
Dose mapping (freestanding irradiators) | Routinely, once a year (137Cs) or twice a year (60Co) and after major repairs, using a fully filled canister (water/plastic) with a dosimetry system to map the distribution of the absorbed dose |
Dose mapping (linear accelerators) | Yearly dose mapping with an ionization chamber and a water phantom recommended; more frequent evaluation of instrument conditions to ensure consistency of X-rays |
Correction for radio isotopic decay | With137 Cs as the source, annually with60 Co as the source, quarterly |
Turntable rotation (freestanding 137 Cs irradiators) | Daily verification |
Storage time for red blood cells after irradiation | For up to 28 days; total storage time cannot exceed the maximum storage time for unirradiated red blood cells |
Storage time for platelets after irradiation | No change related to irradiation procedure |
Parameters that can be monitored after irradiation of blood components for quality control | Hematocrit, plasma Na+, K+levels, plasma LDH, pHb levels also called as supernatant Hb, %Hemolysis of unit (≤0.8% or 1%) |
Although the guidelines are established, adherence to those guidelines in clinical practice is challenging, especially in developing countries. In a recent study from a tertiary hospital, the audit revealed that 7.7 % of units were unnecessarily irradiated and 16% of the requisitions for irradiated blood components were missed though indicated. They emphasized that every center should follow the guidelines with amendments. They should have their own standard operating procedure (SOP) based on review of practices and regular training of medical/paramedical staff to get them sensitized on these safe transfusion practices. Thus, regular training of medical and paramedical personnel is needed. Most of the institutes practice induction programs for their staff to spread awareness of transfusion practices. Each institute and blood center should have an SOP to follow up.[65]
Future perspectives
The potential alternatives to prevent TA-GvHD include depletion of the lymphocytes from blood products or the ultraviolet (UV) irradiation of the blood products. Since the precise number of T-cells required for mediating TA- GvHD is still unknown; therefore, it is unknown whether the depletion of lymphocytes using available techniques will reduce the chances of TA-GvHD. UV blood irradiation can reduce alloimmunization. Future studies are required to find that UV irradiation reduces the risk of TA-GvHD. Recent research methods of lymphocyte inactivation under experiments for TA-GVHD prophylaxis include UVB irradiation and photochemical treatment with UVA-activated psoralens. Psoralens have been developed for pathogen inactivation in platelet and plasma units. There is a hope that it will be helpful in preventing lymphocyte response to immunologic stimulation and proliferation, and work is being evaluated in clinical trials.[66-68]
Irradiation of blood components using UV rays is one of the practiced techniques to prevent transmission of leucotrophic viruses, termed pathogen reduction techniques.[69] There is a need to conduct more studies on the effectiveness of it on a varied spectrum of pathogenic microbes and its safety to use blood for transfusion purposes.
CONCLUSION
Blood irradiation is performed for various clinical indications to reduce the risk of TA-GvHD. One should be aware of the benefits and adverse effects of the same in selecting potential candidates as recipients for irradiated blood products. The available blood irradiations use gamma and X-ray. Gamma irradiators use the source Cesium-137 or Cobalt-60. The blood irradiators are divided broadly into self-contained and panoramic irradiators. The recommended dose for the gamma irradiation is between 15 and 25 Gy with a maximal limit of 50 Gy. The various dosimetry techniques include TLDs, MOSFETs, chloroform/dithizone mixture, alkaline/ESR, and radio-chromic films. There is some degree of uncertainty or error in dose value when one uses different types of dosimetry methods and the value of the uncertainty. Irradiation facility should be available in all transfusion services, if possible, especially those catering to transplant units and tertiary management of clinical condition. Government should support BTS in establishing these facilities to get those services in a timely fashion without delay. There could be some deleterious effects of irradiation on blood components in form of change in electrolytes levels, reduces shelf life, cell membrane damage, theoretical leaching of plasticizers from blood bags to a minute extent, etc. One should keep in mind about this phenomenon in issuing and transfusing patients, especially in morbid cases. Various others mean of preventing and treating GvHD is available as options.
Acknowledgments
The corresponding author AGB is thankful to the Director, Dean-Academics, Dean-Research Cell, and I/c HOD Dept. of Transfusion Medicine and Hemotherapy, All India Institute of Medical Sciences, Mangalagiri, Andhra Pradesh, India, for their encouragement and support.
Author contributions
AB: Concept, design, definition of Intellectual content, literature search, data acquisition and analysis, manuscript preparation, and editing. DSCK: Concept, manuscript review, and editing. ÚM: Literature search and manuscript review.
Ethical approval
The Institutional Review Board approval is not required.
Declaration of patient consent
Patient’s consent was not required as there are no patients in this study.
Conflicts of interest
There are no conflicts of interest.
Use of artificial intelligence (AI)-assisted technology for manuscript preparation
The authors confirm that there was no use of artificial intelligence (AI)-assisted technology for assisting in the writing or editing of the manuscript and no images were manipulated using AI.
Financial support and sponsorship
Nil.
References
- Graft-versus-host reaction following blood product transfusion. Am J Med. 1972;72:951-61.
- [CrossRef] [Google Scholar]
- Non-infectious complications related to blood transfusion: An 11 year retrospective analysis in a tertiary care hospital. Indian J Hematol Blood Transfus. 2016;32:292-8.
- [CrossRef] [Google Scholar]
- Transfusion-associated graft-versus-host disease in immunocompetent patients: Report of a fatal case associated with transfusion of blood from a second-degree relative, and a survey of predisposing factors. Transfusion. 1993;33:742-50.
- [CrossRef] [Google Scholar]
- National healthcare safety network biovigilance component haemovigilance module surveillance protocol. 2018. Available from: https://www.cdc.gov/nhsn/pdfs/biovigilance/bv-hv-protocol-current.pdf [Last accessed on 2023 Dec 21]
- [Google Scholar]
- Stem cell or bone marrow transplant side effects. Available from: https://wwwcancer.org [Last accessed on 2020 Sep 01]
- [Google Scholar]
- Kinetics of bilirubin and liver enzymes is useful for predicting of liver graft-versus-host disease. Neoplasma. 2012;59:264-8.
- [CrossRef] [Google Scholar]
- Cutaneous graft versus host disease in pediatric multivisceral transplantation. Pediatr Dermatol. 2013;30:335-41.
- [CrossRef] [Google Scholar]
- The effect of HLA mismatch in graft-versus-host disease. Int J Hematol. 2013;98:300-8.
- [CrossRef] [Google Scholar]
- GVHD prophylaxis plus ATLG after myeloablative allogeneic haemopoietic peripheral blood stem¬cell transplantation from HLA-identical siblings in patients with acute leukaemia in remission: Final results of quality of life and long-term outcome analysis of a phase 3 randomised study. Lancet Haematol. 2019;6:e89-99.
- [CrossRef] [Google Scholar]
- Immunological considerations for embryonic and induced pluripotent stem cell banking. Philos Trans R Soc Lond B Biol Sci. 2011;366:2312-22.
- [CrossRef] [Google Scholar]
- Acute graft versus host disease: A comprehensive review. Anticancer Res. 2017;37:1547-55.
- [CrossRef] [Google Scholar]
- Regulatory T-cell immunotherapy for tolerance to self-antigens and allo-antigens in humans. Nat Rev Immunol. 2007;7:585-98.
- [CrossRef] [Google Scholar]
- Cellular and molecular mechanisms in graft-versus-host disease. J Leukoc Biol. 2016;99:279-87.
- [CrossRef] [Google Scholar]
- Transfusion-associated graft-versus-host disease. Hum Pathol. 1986;17:1085-8.
- [CrossRef] [Google Scholar]
- Clinical manifestations of graft-versus-host disease in human recipients of marrow from HL-A-matched sibling donors. Transplantation. 1974;18:295-304.
- [CrossRef] [Google Scholar]
- 1994 consensus conference on acute GVHD grading. Bone Marrow Transplant. 1995;15:825-8.
- [Google Scholar]
- IBMTR Severity Index for grading acute graft-versus-host disease: Retrospective comparison with Glucksberg grade. Br J Haematol. 1997;97:855-64.
- [CrossRef] [Google Scholar]
- International, multicenter standardization of acute graft-versus-host disease clinical data collection: A report from the Mount Sinai Acute GVHD International Consortium. Biol Blood Marrow Transplant. 2016;22:4-10.
- [CrossRef] [Google Scholar]
- EBMT-NIH-CIBMTR Task Force position statement on standardized terminology and guidance for graft-versus-host disease assessment. Bone Marrow Transplant. 2018;53:1401-15.
- [CrossRef] [Google Scholar]
- Application of chimerism analysis to allogeneic hematopoietic stem cell transplantation by STR-PCR. Zhongguo Shi Yan Xue Ye Xue Za Zhi. 2007;15:337-41.
- [Google Scholar]
- Acute graft-versus-host disease after liver transplantation diagnosed by fluorescent in situ hybridization testing of skin biopsy specimens. J Am Acad Dermatol. 2006;55:642-6.
- [CrossRef] [Google Scholar]
- The eGVHD App has the potential to improve the accuracy of graft-versus-host disease assessment: A multicenter randomized controlled trial. Haematologica. 2018;103:1698-707.
- [CrossRef] [Google Scholar]
- Transfusion-associated graft-versus-host disease: A concise review. Hematol Rep. 2018;10:7724.
- [CrossRef] [Google Scholar]
- Use of irradiated blood components: Practice parameter. Am J Clin Pathol. 1996;106:6-11.
- [CrossRef] [Google Scholar]
- Guidelines on the use of irradiated blood components. Br J Haematol. 2020;191:704-24.
- [CrossRef] [Google Scholar]
- Association of anaesthetists of great Britain and Ireland: Safe vascular access 2016. Anaesthesia. 2016;71:573-85.
- [CrossRef] [Google Scholar]
- Technical manual United States: American Association of Blood Banks (AABB); 2014. p. :216.
- [Google Scholar]
- Available from: https://www.remm.hhs.gov>blood/transfusion [Last accessed on 2022 Aug 01]
- Biology of graft-versus-host disease. Am J Pediatr Hematol Oncol. 1993;15:18-37.
- [CrossRef] [Google Scholar]
- The clinical significance of human leukocyte antigen (HLA) allele compatibility in patients receiving a marrow transplant from serologically HLA-A, HLA-B, and HLA-DR matched unrelated donors. Blood. 2002;99:4200-6.
- [CrossRef] [Google Scholar]
- Unrelated donor hematopoietic cell transplantation: Marrow or umbilical cord blood? Blood. 2003;101:4233-44.
- [CrossRef] [Google Scholar]
- Hematopoietic engraftment and survival in adult recipients of umbilical-cord blood from unrelated donors. N Engl J Med. 2001;344:1815-22.
- [CrossRef] [Google Scholar]
- A prospective randomized trial comparing cyclosporine/methotrexate and tacrolimus/sirolimus as graft-versus-host disease prophylaxis after allogeneic hematopoietic stem cell transplantation. Haematologica. 2016;101:1417-25.
- [CrossRef] [Google Scholar]
- Mesenchymal stromal cells as treatment or prophylaxis for acute or chronic graft-versus-host disease in haematopoietic stem cell transplant (HSCT) recipients with a haematological condition. Cochrane Database Syst Rev. 2019;1:CD009768.
- [CrossRef] [Google Scholar]
- Control of graft-versus-host disease and graft rejection by T cell depletion of donor and recipient with Campath-1 antibodies. Results of matched sibling transplants for malignant diseases. Bone Marrow Transplant. 1994;13:597-611.
- [Google Scholar]
- Acute graft-vs-host disease: Pathobiology and management. Exp Hematol. 2001;29:259-77.
- [CrossRef] [Google Scholar]
- Intraoral psoralen ultraviolet A irradiation (PUVA) treatment of refractory oral chronic graft-versus-host disease following allogeneic stem cell transplantation. Bone Marrow Transplant. 2001;28:807-8.
- [CrossRef] [Google Scholar]
- Calcineurin is a common target of cyclophilin-cyclosporin A and FKBP-FK506 complexes. Cell. 1991;66:807-15.
- [CrossRef] [Google Scholar]
- Graft versus host disease treatment and management: Medical care. Medscape. Available from: https://emedicine.medscape.com/article/429037-treatment [Last accessed on 2023 Dec 15]
- [Google Scholar]
- Ibrutinib in steroid-refractory chronic graft-versus-host disease, a single-center experience. Transplant Cell Ther. 2021;27:990.e1-7.
- [CrossRef] [Google Scholar]
- World's first stem-cell drug approval achieved in Canada. United States: Drinker Biddle and Reath LLP; Available from: https://natlawreview.com/article [Last accessed on 2023 Dec 15]
- [Google Scholar]
- Increased survival using MSB cells in children with aGVHD. Available from: https://announcements.asx.com.au/asxpdf/20160222/pdf/4356w6pqw4tbmm.pdf
- [Google Scholar]
- The Grading of recommendations, assessment, development, and evaluation approach was rarely followed and inconsistently applied in pressure injury prevention guidelines development: A cross¬sectional survey. J Tissue Viability. 2022;31:438-43.
- [CrossRef] [Google Scholar]
- Available from: http://www.gradeworkinggroup.com [Last accessed on 2022 Aug 01]
- Prevention of transfusion associated graft-versus-host disease: Selection of an adequate dose of gamma irradiation. Transfusion. 1993;33:125-7.
- [CrossRef] [Google Scholar]
- Rossi's principles of transfusion medicine United States: John Wiley and Sons; 2022.
- [CrossRef] [Google Scholar]
- U.S. Food and Drug Administration, Center for Biologics Evaluation and Research. Available from: https://www.fda.gov/cber/guidelines.htm [Last accessed on 2022 Aug 01]
- [Google Scholar]
- Deciding between an X-Ray and 137Cs Irradiator-It's not just about Energy Spectra. Radiat Res. 2019;192:493-506.
- [CrossRef] [Google Scholar]
- Self-contained gamma irradiators (categories I and III) Austria: International Atomic Energy Agency; 1996.
- [Google Scholar]
- Manual on Panoramic Gamma Irradiators Austria: International Atomic Energy Agency; 1996.
- [Google Scholar]
- Compendium of biomedical instrumentation Vol. 3 United States: John Wiley and Sons; 2020.
- [CrossRef] [Google Scholar]
- X-ray radiation as an alternative to gamma radiation for irradiation of blood components. Transfusion. 2000;40:157-158.
- [Google Scholar]
- Absorption of gamma-radiation in simulated blood components using cesium (CS) irradiators. Transfusion. 1995;35:S250.
- [Google Scholar]
- Dosimetry measurements after gamma-irradiation with cesium-137 and linear acceleration sources. Transfusion. 1993;33:S52.
- [Google Scholar]
- Guide to the preparation, use and quality assurance of blood components: Recommendation No R (95) 15 New York: Manhattan Publishing Company; 1997.
- [Google Scholar]
- Deformability and viability of irradiated red cells. Ann Ist Super Sanita. 2007;43:176-85.
- [Google Scholar]
- The effect of pre-storage irradiation on post-transfusion red cell survival. Transfusion. 1992;32:525-8.
- [CrossRef] [Google Scholar]
- Kinetics of extracellular potassium concentration in irradiated red blood cells. Infusion Ther Transfusion Med. 1994;21:322-4.
- [CrossRef] [Google Scholar]
- Viability and in vitro properties of AS-1 red cells after gamma irradiation. Transfusion. 1999;39:128-34.
- [CrossRef] [Google Scholar]
- Radiation-induced hepatitis B virus reactivation in liver mediated by the bystander effect from irradiated endothelial cells. Clin Cancer Res. 2007;13:851-7.
- [CrossRef] [Google Scholar]
- Flowcytometry analysis of gamma-radiation-induced Epstein-Barr virus reactivation in lymphocytes. Radiat Res. 2003;159:268-73.
- [CrossRef] [Google Scholar]
- The effects of irradiationon platelet function. Transfusion. 1988;28:451-5.
- [CrossRef] [Google Scholar]
- The irradiation of blood and blood components to prevent graft-versus-host disease: Technical issues and guidelines. Transfus Med Rev. 1997;11:15-26.
- [CrossRef] [Google Scholar]
- Demographics and appropriateness of cellular blood component irradiation practices: Ambispective analysis from a tertiary care center. Transfus Apher Sci. 2023;62:103651.
- [CrossRef] [Google Scholar]
- Ultraviolet irradiation modulates MHC-alloreactive cytotoxic T-cell precursors involved in the onset of graft-versus-host disease. Br J Haematol. 1992;81:73-6.
- [CrossRef] [Google Scholar]
- Prevention of transfusion-associated graft-versus-host disease by photochemical treatment. Blood. 1999;93:3140-7.
- [CrossRef] [Google Scholar]
- Assessment of donor T-cell function in cellular blood components by the CD69 induction assay: Effects of storage, gamma radiation, and photochemical treatment. Transfusion. 2000;40:761-70.
- [CrossRef] [Google Scholar]
- Antimicrobial 405 nm violet-blue light treatment of ex vivo human platelets leads to mitochondrial metabolic reprogramming and potential alteration of Phospho-proteome. J Photochem Photobiol B. 2023;241:112672.
- [CrossRef] [Google Scholar]