Translate this page into:
The role of erythropoietin in the treatment of ocular disorders: A literature review
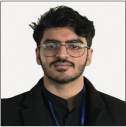
*Corresponding author: Hashim Mohamed Siraj, Department of Medicine, Ivane Javakhishvili Tbilisi State University, Tbilisi, Georgia. hashimmohamedsiraj@gmail.com
-
Received: ,
Accepted: ,
How to cite this article: Mohamed Siraj H. The role of erythropoietin in the treatment of ocular disorders: A literature review. Med India. 2024;3:58-63. doi: 10.25259/MEDINDIA_7_2024
Abstract
Erythropoietin (EPO), traditionally known for its role in hematopoiesis, has garnered increasing attention for its neuroprotective properties, particularly in ocular disorders. This literature review explores the diverse roles of EPO in ocular health and its potential therapeutic applications. EPO’s involvement in ocular organogenesis and maintenance is highlighted, with evidence suggesting its correlation with gestational age and expression alongside crucial regulatory genes during eye development. Pre-clinical studies demonstrate EPO’s efficacy in preserving retinal integrity and mitigating neuronal damage in animal models of glaucoma, methanol optic neuropathy, and optic neuritis. Clinical investigations further support these findings, showing improvements in visual acuity and retinal nerve fiber layer thickness in patients treated with EPO. However, challenges such as optimizing dosage regimens and determining the most effective route of administration remain. Despite these challenges, EPO presents a promising avenue for vision preservation and the management of ocular disorders. Further research, including large-scale clinical trials and mechanistic studies, is warranted to solidify EPO’s role as a safe and effective therapeutic option in ocular diseases.
Keywords
Erythropoietin
Ocular disorders
Glaucoma
Optic neuritis
Methanol optic neuropathy
Retinal protection
INTRODUCTION
Erythropoietin (EPO) is a type of glycoprotein that weighs 30.4 kDa and is primarily made in the liver of fetuses and adult kidneys.[1] While it is mainly known for its role in creating blood cells, it is also created in limited amounts in the central nervous system (CNS), where its receptor, EPO receptor (EPOR), is found in a variety of CNS cells, including neurons, astrocytes, and microglia.[1] About 10% of EPO in the body comes from sources outside of the kidneys.[2] In the past, EPO has been used to increase red blood cell production and treat anemia.[3] In addition, its widespread effects on the body, such as promoting blood vessel growth, reducing inflammation, and stabilizing endothelial cells, have been well-documented.[4]
Recent research has brought to light the potential neuroprotective and neurotrophic abilities of EPO; however, the exact mechanisms are not yet fully understood.[5,6] EPO has shown the ability to decrease apoptosis, reactive oxygen species, excitotoxicity, and inflammation while also promoting the proliferation of progenitor cells.[4,7,8] By regulating apoptosis factors such as Bcl-2-associated X (Bax), Bad, and Bcl-2/Bcl-xL, EPO prevents the formation of the Bax/Bcl complex and reduces the activation of caspases.[9,10] In addition to its potential in treating stroke, EPO also shows promise in addressing delayed degenerative neurological disorders such as amyotrophic lateral sclerosis, Parkinson’s, and Alzheimer’s disease.[11,12] Emerging evidence suggests that EPO plays a significant role in protecting the retina, as well as the CNS, as both EPO and its receptor are present in retinal tissue.[13,14]
This literature review aims to dissect the expanding body of research surrounding the therapeutic effects of EPO, specifically within the realm of ocular pathology. Recent investigations have unveiled promising outcomes with systemic and intravitreal administration of EPO in various ocular conditions, shedding light on its therapeutic potential in retarding degenerative processes and promoting tissue regeneration. By synthesizing findings from both animal models and clinical studies, this review seeks to provide a comprehensive understanding of EPO’s mechanisms of action and its impact on ocular health.
METHODS
The data for this literature review were obtained through a comprehensive search of databases, including PubMed, Google Scholar, and CrossRef. Keywords and Medical Subject Headings terms used in the search strategy included “Erythropoietin,” “EPO,” “Ocular disorders,” “glaucoma,” “optic neuritis,” “methanol optic neuropathy,” and “retinal protection.” The search was limited to articles published in English. Articles were screened based on relevance to the role of EPO in ocular disorders, with a focus on its neuroprotective effects and therapeutic potential. Both experimental studies in animal and clinical trials involving human participants were included. Data extracted from studies included information on study design, intervention details, outcomes measured, and key findings related to EPO’s efficacy and safety. A total of 54 articles were included in this study.
DISCUSSION
The biological role of EPO in the eye
EPO plays a significant role in pre-birth visual function development, which relies on the coordinated activity of regulatory genes that determine cell fate across different origins.[15] Recent research has indicated a direct relationship between fetal retina EPO messenger RNA levels in the vitreous humor and gestational age, highlighting EPO’s involvement in eye development.[15] Studies by Wu et al. further support this notion, revealing the expression of EPO, along with other key proteins such as pro-apoptotic protein Bax, signal transducer activator-of-transcription -5, and EPOR, throughout lens and retinal development.[16] These findings suggest that EPO’s presence during eye organogenesis may contribute to its protective function against cell apoptosis.[16]
The differentiation of ocular progenitor cells into specific retinal neuron types is crucial for proper eye development.[15] Building on previous research, studies have identified EPO’s involvement in neural retina differentiation.[15,16] In addition, EPO may aid in this process by binding to a heterodimer complex comprising EPOR and the interleukin beta-common receptor (βcR; CD131).[17] Recent evidence shows expression of this heterodimer complex in various retinal cell types, including photoreceptor cells, inner nuclear layer, and retinal ganglion cells (RGC).[17] Notably, βcR expression has been detected in eye stem cells, indicating EPO’s potential interaction with the EPOR/βcR complex.[17] Activation of this complex by EPO binding has been shown to stimulate the Wingless (Wnt) signaling pathway, which plays a critical role in eye field, lens, and retina developmental processes.[18-20]
Effects of EPO on glaucoma
EPO, traditionally known for its role in blood cell production, has garnered attention for its tissue-protective properties in pre-clinical models of neurodegeneration, which has spurred interest in exploring EPO’s potential as a therapeutic agent for neuropathic eye conditions like glaucoma.[21] Initial findings in animal models show promise, but further research is required to comprehensively assess the safety and effectiveness of EPO as a neuroprotective treatment in clinical settings.[21]
In their study, Tsai et al. proved that administering EPO directly into the eye can help preserve RGCs in rats with glaucoma caused by cautery of episcleral vessels.[22] A single dose of EPO injected into the eye was effective in reducing the loss of RGCs caused by pressure, indicating its potential as a neuroprotective treatment for glaucoma.[22] Supporting this idea, Junk et al. demonstrated that EPO can also protect retinal neurons from damage caused by acute ischemiareperfusion injury in rats.[23] Administration of recombinant human EPO before or immediately after retinal ischemia resulted in less damage to the tissue and improved function.[23] This was attributed to EPO’s ability to decrease apoptosis in the affected retina, highlighting its anti-apoptotic mechanism of action.[23]
Several studies have shown that patients with various forms of glaucoma, such as acute angle-closure glaucoma, chronic angle-closure glaucoma, primary open-angle glaucoma (POAG), and neovascular glaucoma (NVG), have higher levels of EPO in their aqueous humor.[24,25] This has been confirmed by analyzing aqueous samples from glaucomatous eyes, which consistently show elevated levels of EPO compared to control eyes, regardless of intraocular pressure (IOP) control.[24,25] Interestingly, the correlation between EPO levels and IOP is stronger in pseudoexfoliative glaucoma compared to POAG or NVG.[26] However, there is conflicting evidence on the relationship between EPO levels in the aqueous humor and serum in patients with pseudoexfoliation syndrome and pseudoexfoliative glaucoma.[27]
In a single study, the concentrations of EPO and sCD44 in both the aqueous humor and plasma were investigated in individuals with POAG, and the results indicated significantly increased levels of both biomarkers in POAG patients compared to those in the control group.[28] Furthermore, a strong positive correlation was observed between EPO and sCD44 levels in the aqueous humor of POAG patients.[28] In addition, a noteworthy connection was noted between the levels of EPO or sCD44 and the severity of visual field impairment.[28] Despite previous beliefs of sCD44 as a potentially harmful protein, the authors proposed that the elevated levels of EPO and sCD44 in the aqueous humor of POAG patients may be a consequence of glaucoma-related damage rather than a causative factor.[28]
Effects of EPO on methanol optic neuropathy
Methanol ingestion can lead to severe and irreversible optic neuropathy and neurological impairments.[29] Consumption of illicit alcoholic beverages containing elevated methanol levels presents a substantial risk, as exposure can occur through ingestion, skin contact, or inhalation.[30,31]
In a study conducted between November 2010 and January 2013, individuals experiencing vision loss due to methanol toxicity were recruited.[32] Diagnosis of acute methanol optic neuropathy was based on a decline in visual acuity (VA) within 24–48 h post-ingestion, confirmed by toxicologist reports of methanol intoxication.[32] Eligible participants underwent hospitalization, comprehensive physical examinations, and hematologic assessments, receiving intravenous human recombinant EPO (20,000 international units) for 3 consecutive days.[32] Sixteen participants, with a mean age of 34.2 years, were enrolled.[32] All subjects reported alcohol consumption within 24 h before symptom onset.[32] Before EPO treatment, the median VA in the better eye was light perception (3.60 logMAR, range: 3.90–0.60 logMAR).[32] Following treatment, the median VA in the better eye improved to 1.00 logMAR (range: 3.90–0.00 logMAR).[32] Due to partial improvement after the initial dose, fourteen patients received multiple courses of intravenous treatment.[32] Optical coherence tomography (OCT) data from six patients over a 3-month follow-up period revealed no significant correlation between final VA and peripapillary nerve fiber layer thickness.[32] However, notable developments were observed in two patients during treatment.[32] One patient experienced a slight decline in VA 3 months post-treatment despite initial improvement, while another developed ataxia, tremor, and muscle rigidity, with brain magnetic resonance imaging showing putaminal abnormalities.[32]
Histopathological findings in individuals affected by methanol toxicity suggest demyelination in the retrobulbar optic nerve segment due to the histotoxic effects of formic acid on oxidative metabolism.[33,34] This phenomenon stemsfrom formic acid’s impact on oxidative metabolism in critical regions supplied by cerebral and optic nerve circulations, known as watershed areas.[35,36] Here, formic acid impedes oxidative metabolism by binding to the ferric iron moiety with high affinity, thus hindering the activity of cytochrome C oxidase in mitochondria.[35,36]
Muller cells and photoreceptor dysfunction also contribute to methanol toxicity, for which treatment involves correcting metabolic acidosis, eliminating methanol through hemodialysis, and inhibiting toxic metabolite formation.[37,38] EPO, initially identified as a hematopoietic growth factor, exhibits pleiotropic properties, which could contribute to its beneficial effects in this context.[39]
EPO promotes RGC and axon survival, enhances growth-associated protein-43 expression, and protects against glutamate-induced cytotoxicity and nitric oxide toxicity by increasing Bcl-2 expression.[40] In addition, EPO mitigates oxidative stress-induced retinal pigment epithelial cell apoptosis and exerts neuroprotective effects on CNS tissues.[40] Studies have demonstrated the potential efficacy of EPO in safeguarding neural tissues following metabolic stress.[41] Furthermore, it may play a role in protecting or enhancing the function of dopaminergic neurons in the substantia nigra.[42,43]
Intravenous EPO demonstrates rapid VA improvement within 3 weeks post-methanol ingestion, suggesting its potential as a treatment modality.[32] However, limitations, including lack of a control group, insufficient visual field data, and small sample size, warrant further clinical trials to determine optimal dosage, timing, and structural effects on the optic nerve.[32]
Effects of EPO on optic neuritis
The TONE experiment, a multicenter trial with two parallel arms, was conducted to investigate the effectiveness of EPO treatment for optic neuritis (ON), a common symptom of multiple sclerosis (MS) that leads to degeneration of the optic nerve and retina.[44] The study, which began in September 2014 and ended in February 2016, enrolled 51 patients from various centers.[44] While methylprednisolone has been shown to speed up visual recovery in ON, it does not have a significant impact on visual outcomes, lesion length, or optic nerve atrophy.[45] However, animal studies have suggested that methylprednisolone may actually worsen RGC degeneration.[46] Furthermore, research on optic neuritis in animals has revealed that the mechanisms of neurodegeneration involve pathways regulated by neurotrophins.[47]
The TONE trial was based on the VISION PROTECT regimen, which had one arm receiving a daily dose of 33,000 IU of human recombinant EPO for 3 days and the other arm receiving a placebo along with 100 mg of intravenous methylprednisolone for 3 days.[48] Regardless of gender, patients with acute ON were included in the study with written informed consent.[44] The administration of EPO was deemed experimental, while the placebo was used as the control intervention.[44] The study aimed to measure two primary endpoints: The average thickness of the retinal nerve fiber layer (RNFL) in all retinal segments and the low contrast VA (LCVA) in the affected eye 6 months after randomization. [44] Using time-domain OCT, researchers were able to observe a thinning of the RNFL from 100 µm to 78 µm in 74% of patients with ON within a 6-month period.[49]
Several investigations have demonstrated the reliability of OCT in measuring RNFLT and determining the level of optic nerve atrophy, particularly after ON.[44] The advancement of spectral-domain OCT has further improved the accuracy of measuring RNFL thickness.[44]
The primary outcome included LCVA in the affected eye after 6 months, as LCVA strongly correlates with health-related quality of life and RNFLT in patients with MS post-ON.[48,50,51] The study also had secondary objectives, which involved using seven different OCT analyses to investigate any potential neuroprotective effects.[44] The sample size was determined based on the RNFLT-G-12, with the expectation that there would be a difference of 10 µm in RNFLT-G-12, similar to previous findings from the VISION PROTECT study.[52]
Statistical analyses employed hierarchical testing procedures to maintain a type I error of α= 5%.[44] An interim analysis was planned after randomizing 50 patients, focusing on patient recruitment, treatment compliance, and safety.[53] Results were reported only to the independent data monitoring committee, which oversaw study progress and provided recommendations regarding study continuation or modification.[44] The study underscores the collaborative effort between neurologists and ophthalmologists, emphasizing its prospective, double-blind, randomized clinical trial design conducted across various German University Medical Centers.[44] The positive outcomes of the study have implications not only for enhancing ON treatment but also for advancing therapy development in MS, given that RNFL atrophy serves as a surrogate marker for neuronal degeneration in the disease and correlates with overall brain atrophy.[54]
CONCLUSION
EPO exhibits neuroprotective properties that hold promise for treating ocular disorders and neurodegenerative injuries, particularly by mitigating RGC degeneration and safeguarding neurons against mechanical damage. Its involvement in ocular development and maintenance, combined with both preclinical and clinical evidence demonstrating its capacity to preserve retinal integrity and improve visual outcomes, underscores its significance in ophthalmic research. However, several challenges remain, including the need for comprehensive visual data, control groups, and rigorous evaluation of safety and efficacy to establish the effectiveness of EPO. Optimizing dosage, route of administration, and assessing potential adverse effects are also crucial areas requiring further investigation. Nevertheless, EPO’s multifaceted neuroprotective properties offer hope for preserving vision and managing debilitating ocular conditions. To advance its therapeutic potential, large-scale clinical trials and mechanistic studies are essential to confirm EPO’s safety and efficacy in ocular disorders. This would ultimately lead to improved patient outcomes and a better quality of life for those affected by such conditions.
Author contributions
Hashim Mohamed Siraj - Data curation; formal analysis; investigation; methodology; supervision; validation; visualization; writing – review & editing, accountability.
Ethical approval
Institutional Review Board approval is not required.
Declaration of patient consent
Patient’s consent is not required as there are no patients in this study.
Conflicts of interest
There are no conflicts of interest.
Use of artificial intelligence (AI)-assisted technology for manuscript preparation
The authors confirm that they have used artificial intelligence (AI)-assisted technology to improve the readability of the manuscript.
Financial support and sponsorship
Nil.
References
- Erythropoietin and the nervous system. Brain Res. 2004;1000:19-31.
- [CrossRef] [PubMed] [Google Scholar]
- Erythropoietin protects adult retinal ganglion cells against NMDA-, trophic factor withdrawal-, and TNF-α-induced damage. PLoS One. 2013;8:e55291.
- [CrossRef] [PubMed] [Google Scholar]
- An update on the cardiac effects of erythropoietin cardioprotection by erythropoietin and the lessons learnt from studies in neuroprotection. Cardiovasc Res. 2004;63:208-16.
- [CrossRef] [PubMed] [Google Scholar]
- EPO Mediates neurotrophic, neuroprotective, anti-oxidant, and anti-apoptotic effects via downregulation of miR-451 and miR-885-5p in SH-SY5Y neuron-like cells. Front Immunol. 2014;5:475.
- [CrossRef] [PubMed] [Google Scholar]
- Erythropoietin and the brain: From neurodevelopment to neuroprotection. Clin Sci (Lond). 2002;103:275-82.
- [CrossRef] [PubMed] [Google Scholar]
- Erythropoietin in the central nervous system, and its use to prevent hypoxic-ischemic brain damage. Acta Paediatr Suppl. 2002;91:36-42.
- [CrossRef] [PubMed] [Google Scholar]
- Brain and cancer: The protective role of erythropoietin. Med Res Rev. 2005;25:245-59.
- [CrossRef] [PubMed] [Google Scholar]
- Erythropoietin: New directions for the nervous system. Int J Mol Sci. 2012;13:11102-29.
- [CrossRef] [PubMed] [Google Scholar]
- Erythropoietin as an antiapoptotic, tissue-protective cytokine. Cell Death Differ. 2004;11(Suppl 1):S37-44.
- [CrossRef] [PubMed] [Google Scholar]
- Erythropoietin receptor signaling is required for normal brain development. Development. 2002;129:505-16.
- [CrossRef] [PubMed] [Google Scholar]
- Erythropoietin-mediated neuroprotection involves cross-talk between Jak2 and NF-nB signalling cascades. Nature. 2001;412:641-7.
- [CrossRef] [PubMed] [Google Scholar]
- Erythropoietin crosses the blood-brain barrier to protect against experimental brain injury. Proc Natl Acad Sci U S A. 2000;97:10526-31.
- [CrossRef] [PubMed] [Google Scholar]
- Expression pattern of erythropoietin and erythropoietin receptor in experimental model of retinal detachment. Curr Eye Res. 2007;32:757-64.
- [CrossRef] [PubMed] [Google Scholar]
- Elevated erythropoietin mRNA and protein concentrations in the developing human eye. Pediatr Res. 2008;63:394-7.
- [CrossRef] [PubMed] [Google Scholar]
- Erythropoietin receptor plays a role in the cell differentiation of retina and lens during eye development. Invest Ophthalmol Vis Sci. 2008;49:3078.
- [CrossRef] [PubMed] [Google Scholar]
- Non-erythropoietic erythropoietin derivatives protect from light-induced and genetic photoreceptor degeneration. Hum Mol Genet. 2011;20:2251-62.
- [CrossRef] [PubMed] [Google Scholar]
- Evidence that erythropoietin modulates neuroinflammation through differential action on neurons, astrocytes, and microglia. Front Immunol. 2014;5:523.
- [CrossRef] [Google Scholar]
- Dkk1-mediated inhibition of Wnt signaling in bone results in osteopenia. Bone. 2006;39:754-66.
- [CrossRef] [PubMed] [Google Scholar]
- WNT/Frizzled signaling in eye development and disease. Front Biosci. 2006;11:2442-64.
- [CrossRef] [PubMed] [Google Scholar]
- Erythropoietin: A candidate neuroprotective agent in the treatment of glaucoma. J Glaucoma. 2007;16:567-71.
- [CrossRef] [PubMed] [Google Scholar]
- Intravitreal administration of erythropoietin and preservation of retinal ganglion cells in an experimental rat model of glaucoma. Curr Eye Res. 2005;30:1025-31.
- [CrossRef] [PubMed] [Google Scholar]
- Erythropoietin administration protects retinal neurons from acute ischemia-reperfusion injury. Proc Natl Acad Sci U S A. 2002;99:10659-64.
- [CrossRef] [PubMed] [Google Scholar]
- Erythropoietin is increased in aqueous humor of glaucomatous eyes. Curr Eye Res. 2010;35:680-4.
- [CrossRef] [PubMed] [Google Scholar]
- Aqueous humor erythropoietin levels in patients with primary open-angle glaucoma. J Glaucoma. 2007;16:645-8.
- [CrossRef] [PubMed] [Google Scholar]
- Erythropoietin levels in aqueous humor of patients with glaucoma. Mol Vis. 2012;18:1991-5.
- [Google Scholar]
- Aqueous humor and serum erythropoietin levels in patients with pseudoexfoliation syndrome and pseudoexfoliative glaucoma. Int Ophthalmol. 2010;30:669-74.
- [CrossRef] [PubMed] [Google Scholar]
- Erythropoietin and soluble CD44 levels in patients with primary open-angle glaucoma. Clin Exp Ophthalmol. 2010;38:560-5.
- [CrossRef] [PubMed] [Google Scholar]
- American Academy of Clinical Toxicology practice guidelines on the treatment of methanol poisoning. J Toxicol Clin Toxicol. 2002;40:415-46.
- [CrossRef] [PubMed] [Google Scholar]
- Comparison of methanol exposure routes reported to Texas poison control centers. West J Emerg Med. 2008;9:150-3.
- [Google Scholar]
- Methanol (EHC 196 1997) (n.d.). Available from: https://www.inchem.org/documents/ehc/ehc/ehc196.htm [Last accessed on 2024 May 03]
- [Google Scholar]
- Erythropoietin in treatment of methanol optic neuropathy. J Neuroophthalmol. 2018;38:167-71.
- [CrossRef] [PubMed] [Google Scholar]
- Optic nerve involvement in a case of methanol poisoning. Br J Ophthalmol. 1988;72:778-81.
- [CrossRef] [PubMed] [Google Scholar]
- Methanol optic neuropathy: A histopathological study. Neurology. 1982;32:1093-100.
- [CrossRef] [PubMed] [Google Scholar]
- The ocular effects of methyl alcohol poisoning: report of a catastrophe involving three hundred and twenty persons. Trans Am Acad Ophthalmol Otolaryngol. 1952;56:875-85.
- [Google Scholar]
- Late treatment of methanol blindness. Br J Ophthalmol. 1997;81:416-7.
- [CrossRef] [PubMed] [Google Scholar]
- Methanol poisoning: Ocular toxicity produced by formate. Toxicol Appl Pharmacol. 1978;45:201-8.
- [CrossRef] [PubMed] [Google Scholar]
- Toxic alcohol ingestions: Clinical features, diagnosis, and management. Clin J Am Soc Nephrol. 2008;3:208-25.
- [CrossRef] [PubMed] [Google Scholar]
- Erythropoietin: Physiology and pharmacology update. Exp Biol Med (Maywood). 2003;228:1-14.
- [CrossRef] [PubMed] [Google Scholar]
- The protective effect of erythropoietin on the retina. Ophthalmic Res. 2015;53:74-81.
- [CrossRef] [PubMed] [Google Scholar]
- Erythropoietin prevents neuronal apoptosis after cerebral ischemia and metabolic stress. Proc Natl Acad Sci U S A. 2001;98:4044-9.
- [CrossRef] [PubMed] [Google Scholar]
- Recent trends in erythropoietin-mediated neuroprotection. Int J Dev Neurosci. 2008;26:103-11.
- [CrossRef] [PubMed] [Google Scholar]
- Intrastriatal administration of erythropoietin protect dopaminergic neurons and improves neurobehavioral outcome in a rat model of Parkinson's disease. Neuroscience. 2007;146:1245-58.
- [CrossRef] [PubMed] [Google Scholar]
- Treatment of optic neuritis with erythropoietin (TONE): A randomized, double-blind, placebo-controlled trial-study protocol. BMJ Open. 2016;6:e010956.
- [CrossRef] [PubMed] [Google Scholar]
- Corticosteroids for treating optic neuritis. Cochrane Database Syst Rev. 2015;2015:CD001430.
- [CrossRef] [PubMed] [Google Scholar]
- Methylprednisolone increases neuronal apoptosis during autoimmune CNS inflammation by inhibition of an endogenous neuroprotective pathway. J Neurosci. 2003;23:6993-7000.
- [CrossRef] [PubMed] [Google Scholar]
- Neuroprotective effects and intracellular signaling pathways of erythropoietin in a rat model of multiple sclerosis. Cell Death Differ. 2004;11(Suppl 2):S181-92.
- [CrossRef] [PubMed] [Google Scholar]
- A randomized, controlled trial of corticosteroids in the treatment of acute optic neuritis. The Optic Neuritis Study Group. N Engl J Med. 1992;326:581-8.
- [CrossRef] [PubMed] [Google Scholar]
- Quantifying axonal loss after optic neuritis with optical coherence tomography. Ann Neurol. 2006;59:963-9.
- [CrossRef] [PubMed] [Google Scholar]
- Vision related quality of life in multiple sclerosis: Correlation with new measures of low and high contrast letter acuity. J Neurol Neurosurg Psychiatry. 2009;80:767-72.
- [CrossRef] [PubMed] [Google Scholar]
- Measurement of the retinal nerve fibre layer with scanning laser polarimetry in patients with previous demyelinating optic neuritis. J Neurol Neurosurg Psychiatry. 1998;64:505-9.
- [CrossRef] [PubMed] [Google Scholar]
- A randomized, double-blind, phase 2 study of erythropoietin in optic neuritis. Ann Neurol. 2012;72:199-210.
- [CrossRef] [PubMed] [Google Scholar]
- A serial study of retinal changes following optic neuritis with sample size estimates for acute neuroprotection trials. Brain. 2010;133:2592-602.
- [CrossRef] [PubMed] [Google Scholar]
- Retinal nerve fiber layer is associated with brain atrophy in multiple sclerosis. Neurology. 2007;69:1603-9.
- [CrossRef] [PubMed] [Google Scholar]